Like a Fingerprint
Like a Fingerprint
Engineering an Innovative Approach to Diagnosing Diseases
When we ask our doctors to run tests to diagnose an ailment, we tend to think of glass slides under microscopes. You might be surprised to learn that electrical engineers are creating devices that help clinicians and physicians detect and treat viruses and bacterial infections, as well as chronic conditions such as diabetes.
Nathan Swami, professor of electrical and computer engineering, is motivated by a desire to make medical treatments more precise with better diagnostic systems that medical personnel can use when a patient first walks through the door, even in areas where medical resources are scarce.
With students in his Biophysical Microsystems Group, Swami published 15 papers in 2020; three appeared as cover articles in ACS Infectious Diseases, Lab on a Chip, and ACS Sensors. Seed grants from the UVA Cancer Center and UVA’s Global Infectious Diseases Institute fund his research, supplemented by grant awards from the National Center for Advancing Translational Sciences and Integrated Translational Health Research Institute of Virginia, in addition to a LaunchPad award from the Manning Family Foundation. Swami’s innovations in biofabrication, electrokinetics, and electrochemistry in microfluidic systems earned him the AES Electrophoresis Society’s 2021 Mid-Career Award.
In this Q&A, Swami shares his research methods and perspective on electrical engineers’ contributions to diagnostic tools for precision medicine.
Q. The nation’s COVID-19 response has relied in large part on testing. Even with vaccines’ availability, testing remains an important element in containing the spread of the disease. How might your research help doctors and public health officials track COVID-19 cases?
The most familiar test for COVID-19 is the nasal swab, also called a polymerase chain reaction (PCR) test. It detects genetic material from a specific organism, such as a virus. It then compares the genetic sequence of the nucleic acid—the RNA—inside the cells collected with known RNA sequences of COVID-19. The PCR test returns a straightforward yes-no response, whether you are infected or not.
The nasal swab and other tests of cells’ genetic makeup have their limitations, however. They cannot tell you how infective you are or how long you have been infected.
Our sensing approach overcomes these limitations. Specific to COVID-19, we would look at how the spike protein key opens the lock of a healthy cell. More broadly, we use a cell’s physical characteristics—technically speaking, its phenotype—such as its protein layer, its structure, and its secretions, to measure the number and type of shed virus particles that can bind to host cells. This correlates to the individual’s infectivity.
The exact quantity of the virus is very important to know, especially in situations in which underlying conditions like asthma accentuate COVID’s harm. These individuals will feel the effects of COVID-19 at a lower dose of the virus compared with individuals who are in full health.
We see our approach as a complement to rather than a replacement for COVID-19 PCR-type
tests. On the assumption that COVID-19 variants will be an ongoing public health concern akin to
the seasonal flu, we hope to offer more focused, second-level tests to determine whether a person’s viral load is at or above a threshold that makes the disease dangerous to them or others they
may contact.
Q. Tell us a bit more about how your device works.
We have designed a microchip cartridge onto which we can drop a saliva sample and then utilize an electrical field to separate, detect, and probe single virus particles that are bound to a receptor tagged with a conductive nanostructure probe. The saliva sample will contain many particles; we are detecting them one by one, identifying the particle of interest based on the conductivity tag on its receptor and probing each particle’s interior layers by alternating current fields. This information creates something like a fingerprint.
We can classify the sample according to how many infective virus particles are released over a given period of time. This measurement can tell us how infective a person is.
The good thing about what we are doing is that it doesn’t require sophisticated facilities. It only requires an electric field generation system, which many microchips in cell phones and computers can produce. So it's perfect for point-of-care or resource-poor settings. The beauty of our approach is that it is disease-agnostic. It is designed to be of use in diagnosing a wide variety of viral and bacterial infections as well as chronic diseases.
Top: Biophysical phenotypes of virus particles measured by the microchip at single-cell sensitivity are used to determine their infection ability.
Bottom: Nathan Swami is the principal investigator and directs research for the Biophysical Microsystems Group, or BIOSyS, a team of research scientists and graduate students.
Q. Your analogy to fingerprinting suggests that you need a huge database and machine learning to quickly analyze cells’ physical characteristics. Does that database exist today?
We need data, yes, but we envision a shared network of crowdsourced data repositories rather than a single, centralized database.
We recently published a paper, “Single-cell Microfluidic Impedance Cytometry: From Raw Signals to Cell Phenotypes Using Data Analytics,” selected as a cover article for Lab on a Chip. It presents data analytics for high-throughput phenotypic identification of cells, bacteria, and viruses. The journal is available through MedLine and reaches a wide audience of physicians, clinicians, and lab techs.
We partnered with Paolo Bisegna and Federica Caselli in the department of computer science at the University of Rome. They specialize in machine learning methods applied to the biophysical data that we generate in my lab. I should also mention that my postdoc Carlos Honrado was first author of the paper. We have another paper submitted in collaboration with the Air Force Research Lab that involved Yi Liu, another postdoctoral scientist in my research group, that delves further into the detection paradigm, specifically the selection of the receptor for binding the virus’ surface-layer protein.
We aim to educate the medical community about this new way to measure infectivity based on biomarkers.
Our paper in Lab on a Chip proposes a way to compare apples to apples when looking at two or more samples, that is, to normalize impedance data across measurements and labs. We also encourage doctors and labs to use our sensor in their own context, on a variety of viral and bacterial infections and chronic diseases.
In this way, we can develop a data system and architecture of biophysical models, share the data code, and enable others to write their own code. If we successfully promote this structure to quantify and classify data, we can more easily look for significant patterns such as immune reaction.
As more groups participate in using this data structure, we can relate biomarkers to particular diseases. This is a big leap, however, and it will take a sustained, long-term effort to make it happen. We need to identify the particular chemical and physical characteristics of each cell phenotype of interest and model how it interacts with another cell to understand how a disease-carrying cell affects the whole body. And we would need to repeat this process for each disease.
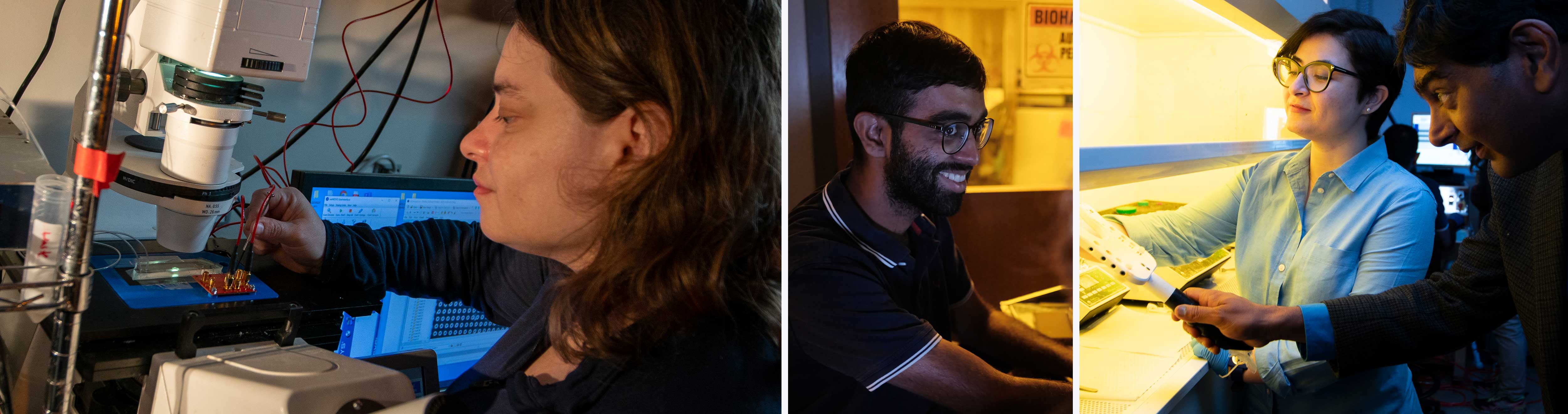
Left: Electrical engineering doctoral student Karina Torres-Castro. Middle: Aditya Rane, doctoral student in chemistry. Right: Electrical engineering doctoral student Armita Salahi with Nathan Swami, professor of electrical and computer engineering.
Q. We like to think of data as empowering patients as well as their doctors. You’ve often expressed your desire to help people independently monitor and assess their medical needs and treatments. Could you say more about this long-term vision?
We want to empower patients so they don’t have to wait on medical professionals to interpret their biophysical metrics and give them direction. Our device and data architecture are a baby step toward a portable way to read and classify biophysical data at the cellular level.
Let’s say you need to monitor immune system performance on a regular basis. You could extract a small blood sample, like a finger prick, put it through a micro device that can quantify white blood cell phenotypes based on immune cell activation and immune reactions due to immune cell activation and immune reactions to transplanted tissues or due to secretions against pathogens. Together, these can indicate whether and how the immune system is breaking down. That’s a long way off, but that’s the vision.